Life on Earth Depends on Networks of Ocean Bacteria – WIRED
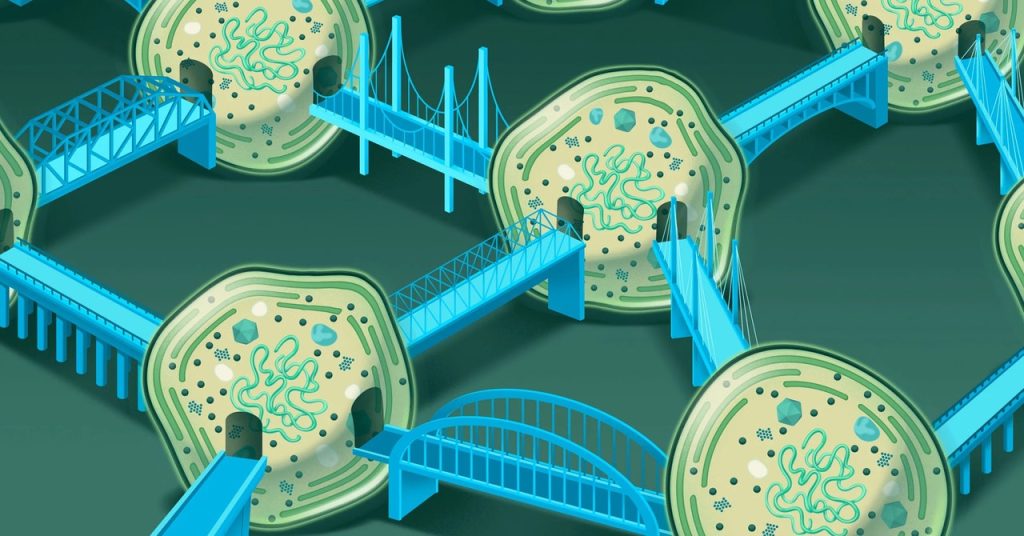
The original version of this story appeared in Quanta Magazine.Prochlorococcus bacteria are so small that you’d have to line up around a thousand of them to match the thickness of a human thumbnail. The ocean seethes with them: The microbes are likely the most abundant photosynthetic organism on the planet, and they create a significant portion—10 percent to 20 percent—of the atmosphere’s oxygen. That means that life on Earth depends on the roughly 3 octillion (or 3 × 1027) tiny individual cells toiling away.Biologists once thought of these organisms as isolated wanderers, adrift in an unfathomable vastness. But the Prochlorococcus population may be more connected than anyone could have imagined. They may be holding conversations across wide distances, not only filling the ocean with envelopes of information and nutrients, but also linking what we thought were their private, inner spaces with the interiors of other cells.At the University of Córdoba in Spain, not long ago, biologists snapping images of the cyanobacteria under a microscope saw a cell that had grown a long, thin tube and grabbed hold of its neighbor. The image made them sit up. It dawned on them that this was not a fluke.“We realized the cyanobacteria were connected to each other,” said María del Carmen Muñoz-Marín, a microbiologist there. There were links between Prochlorococcus cells, and also with another bacterium, called Synechococcus, which often lives nearby. In the images, silvery bridges linked three, four, and sometimes 10 or more cells.Muñoz-Marín had a hunch about the identity of these mysterious structures. After a battery of tests, she and her colleagues recently reported that these bridges are bacterial nanotubes. First observed in a common lab bacterium only 14 years ago, bacterial nanotubes are structures made of cell membrane that allow nutrients and resources to flow between two or more cells.The structures have been a source of fascination and controversy over the last decade, as microbiologists have worked to understand what causes them to form and what, exactly, travels among these networked cells. The images from Muñoz-Marín’s lab marked the first time these structures have been seen in the cyanobacteria responsible for so much of the Earth’s photosynthesis.They challenge fundamental ideas about bacteria, raising questions such as: How much does Prochlorococcus share with the cells around it? And does it really make sense to think of it, and other bacteria, as single-celled?Many bacteria have active social lives. Some make pili, hairlike growths of protein that link two cells to allow them to exchange DNA. Some form dense plaques together, known as biofilms. And many emit tiny bubbles known as vesicles that contain DNA, RNA or other chemicals, like messages in a bottle for whatever cell happens to intercept them.It was vesicles that Muñoz-Marín and her colleagues, including José Manuel García-Fernández, a microbiologist at the University of Córdoba, and graduate student Elisa Angulo-Cánovas, were looking for as they zoomed in on Prochlorococcus and Synechococcus in a dish. When they saw what they suspected were nanotubes, it was a surprise.Growing between these bacteria (left: Prochlorococcus; right: Bacillus subtilis) are nanotube bridges, through which cells transport substances such as amino acids and enzymes. Although these nanotubes were first observed only in 2011, biologists now think that bacteria have been making these structures all along unnoticed.Nanotubes are a recent addition to scientists’ understanding of bacterial communication. In 2011, Sigal Ben-Yehuda and her postdoc Gyanendra Dubey at the Hebrew University of Jerusalem first published images of tiny bridges, made of membrane, between the bacteria Bacillus subtilis. These tubes were actively transporting material: The researchers showed that green fluorescent proteins produced in one cell of the network quickly percolated through the others. They found the same result with calcein, a small molecule that is not able to cross bacterial membranes on its own. These cells were not existing placidly side by side; their inner spaces were linked, more like rooms in a house than detached dwellings.It was a startling revelation. The news compelled other biologists to reexamine their own images of cells. It soon became clear that B. subtilis was not the only species producing nanotubes. In populations of Escherichia coli and numerous other bacteria, small but consistent fractions of cells were spotted with nanotubes. In experiments, scientists watched cells sprout from the tubes and then investigated what they carried. Moving across these bridges from cell to cell were substances such as amino acids, the basic building blocks of proteins, as well as enzymes and toxins. Bacteria, biologists now think, have probably been making these structures all along. Scientists simply hadn’t noticed them or realized their significance.Not everyone has found it straightforward to get bacteria to make nanotubes. Notably, a group at the Czech Academy of Sciences could see nanotubes only when cells were dying. Their suggestion that the tubes are a “manifestation of cell death” cast doubt on whether the structures were truly an important part of the cells’ normal biology. Since then, however, additional work has carefully documented that healthy cells do grow the structures. All this suggests that certain conditions must be met for bacteria to take this step. Still, “I think they are everywhere,” Ben-Yehuda said.The latest findings are particularly eye-opening because Prochlorococcus and Synechococcus are not your average dish-dwelling bacteria. They live in a singularly turbulent environment: the open ocean, where water movement might reasonably be expected to break the fragile tubes. What’s more, they are photosynthetic, meaning that they get most of what they need to survive from the sun. What need could they have for trading through tube networks? There has been another sighting of nanotubes in marine bacteria, but those microbes are not photosynthetic—they gobble up nutrients from their immediate environment, a lifestyle in which swapping substances with neighbors might have a more obvious benefit.So, when Muñoz-Marín and Angulo-Cánovas saw their nanotubes, they were initially skeptical. They wanted to make sure that they weren’t mistaking some accident of how the cells were prepared or how the images had been taken for a natural structure.“We spent a lot of time to ensure that what we were finding in the images was actually something physiological and not any kind of an artifact,” García-Fernández said. “The results were so shocking in the field of marine cyanobacteria that we were, on the one hand, amazed, and on the other hand, we wanted to be completely sure.”They put the cells under four radically different kinds of imaging devices—not only a transmission electron microscope, which they had been using when they first spotted the structures, but also a fluorescence microscope, a scanning electron microscope, and an imaging flow cytometer, which images live cells as they zip by. They looked at Prochlorococcus and Synechococcus on their own and at cultures where they lived together. They looked at dead cells and living ones. They even looked at fresh samples of seawater fished out of the Bay of Cádiz. In all the samples they spotted bridges, which connected about 5 percent of the cells. The nanotubes did not seem to be artifacts.From left: José Antonio González-Reyes, Jesús Díez, María del Carmen Muñoz-Marín, Elisa Angulo-Cánovas and José Manuel García-Fernández, all based at the University of Córdoba. The researchers were part of an interdisciplinary group that discovered and studied the bacterial nanotubes that grow between photosynthetic ocean bacteria.Next, to see whether the links were in fact nanotubes, they performed versions of the now-canonical experiments with green fluorescent protein and calcein described by Ben-Yehuda and Dubey. The networked cells lit up. The team also confirmed that the links were indeed made of membrane lipids and not protein, which would instead suggest pili. They were convinced, finally, that they were looking at bacterial nanotubes.These tubes connect some of the most abundant organisms on the planet, they realized. And that immediately made something very clear, something the researchers are still turning over in their minds.“At the beginning of this century, when you were speaking about phytoplankton in the ocean, you were thinking about independent cells that are isolated,” García-Fernandez said. “But now—and not only from these results, but also from results from other people—I think we have to consider that these guys are not working alone.”There might be a good reason why cyanobacteria, floating in the vast expanse of the ocean, might want to join forces. They have curiously small genomes, said Christian Kost, a microbial ecologist at the University of Osnabrück in Germany who was not involved in this study. Prochlorococcus has the smallest genome of any known free-living photosynthetic cell, with only around 1,700 genes. Synechococcus is not far behind.Among bacteria, small genomes relieve organisms of the pressure of maintaining bulky DNA, but this state also requires them to scavenge many basic nutrients and metabolites from their neighbors. Bacteria with streamlined genomes sometimes form interdependent communities with organisms that produce what they need and need what they produce.“This can be much more efficient than a bacterium that attempts to produce all metabolites at the same time,” Kost said. “Now, the problem, when you’re living in a liquid, is: How do you exchange these metabolites with other bacteria?”Nanotubes may be a solution. Nutrients transferred this way will not be swept away by currents, lost to dilution or consumed by a freeloader. In computer simulations, Kost and his colleagues have found that nanotubes can support the development of cooperation among groups of bacteria.What’s more, “this [new] paper shows that this transfer is both happening within and between species,” he said. “This is super interesting.” In a previous paper, he and colleagues also noticed different species of bacteria connected by nanotubes.This kind of cooperation is probably more common than people realize, said Conrad Mullineaux, a microbiologist at Queen Mary University of London—even in environments like the open ocean, where bacteria may not always be close enough to form nanotubes.We often speak of bacteria as being simple and single-celled. But bacterial colonies, biofilms, and consortiums of different microorganisms can perform complicated feats of engineering and behavior together, sometimes rivaling what multicellular life can achieve. “I like to try to persuade people sometimes, when I’m feeling feisty: You’re a biofilm and I’m a biofilm,” Mullineaux said. If the sea is full of cyanobacteria communicating by nanotube and vesicle, then perhaps this exchange of resources could affect something as fundamental as the amount of oxygen in the atmosphere or the amount of carbon sequestered in the ocean.Kost, Ben-Yehuda, and Mullineaux agree that the new paper’s findings are intriguing. The authors have done all the right tests to ensure that the structures they are seeing are in fact nanotubes, they said. But more work is needed to explain the significance of the finding. In particular, a big open question is what, exactly, Prochlorococcus and Synechococcus are sharing with each other in the wild. Photosynthesis allows these bacteria to draw energy from the sun, but they must pick up nutrients such as nitrogen and phosphorus from the environment. The researchers are embarking on a series of experiments with Rachel Ann Foster of Stockholm University, a specialist in nutrient flow in the ocean, to trace these substances in networked cells.Another question is how bacteria form these tubes, and under what conditions. The tubes are not much longer than an individual cell, and Prochlorococcus, in particular, is thought to spread out in the water column. Muñoz-Marín and her team are curious about the concentrations of bacteria required for a network to form. “How often would it be possible for these independent cells to get close enough to each other in order to develop these nanotubes?” García-Fernandez asked. The current study shows that nanotubes do form among wild-caught cells, but the precise requirements are unclear.Looking back at what people thought about bacterial communication when he began to study marine cyanobacteria 25 years ago, García-Fernandez is conscious that the field has undergone a sea change. Scientists once thought they saw myriad individuals floating alongside each other in immense space, competing with neighboring species in a race for resources. “The fact that there can be physical communication between different kind of organisms—I think that changes many, many previous ideas on how the cells work in the ocean,” he said. It’s a far more interconnected world than anyone realized.Original story reprinted with permission from Quanta Magazine, an editorially independent publication of the Simons Foundation whose mission is to enhance public understanding of science by covering research developments and trends in mathematics and the physical and life sciences.Our latest scoop reveals the young, inexperienced engineers aiding Elon Musk’s government takeoverIn your inbox: Will Knight’s AI Lab explores advances in AINvidia’s $3,000 ‘personal AI supercomputer’Big Story: The school shootings were fake. The terror was realEvent: Join us for WIRED Health on March 18 in LondonMore From WIREDReviews and Guides© 2025 Condé Nast. All rights reserved. WIRED may earn a portion of sales from products that are purchased through our site as part of our Affiliate Partnerships with retailers. The material on this site may not be reproduced, distributed, transmitted, cached or otherwise used, except with the prior written permission of Condé Nast. Ad Choices
Source: https://www.wired.com/story/life-on-earth-depends-on-networks-of-ocean-bacteria/