How Does a Nucleus Get Its Shape? – Physics
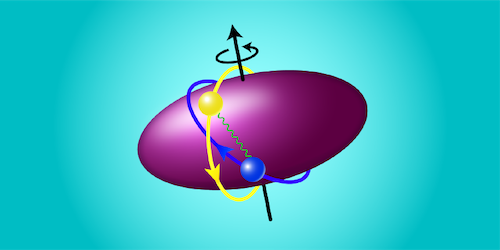
Naively, one would expect a bound state of protons and neutrons to naturally form a spherical shape, as do the electrons in an atom or the molecules in a drop of liquid. In fact, most atomic nuclei are deformed in their ground state [1]. This behavior must be connected to some specific features of the interaction between protons and neutrons. Although much work has qualitatively clarified the mechanisms of nuclear deformation, quantitatively tying deformation to the underlying interaction has been formidably difficult. Now Zhonghao Sun of Oak Ridge National Laboratory, Tennessee, and his collaborators have demonstrated that nuclear theorists are on the cusp of meeting that challenge [2].Nuclei are not the only microscopic systems that adopt nonspherical shapes. A simple example is a diatomic molecule such as H2; the molecule is elongated along the axis connecting the two protons. But analyzing deformation in nuclei provides several additional challenges. First, the Coulomb force that drives molecular physics is much simpler than the interaction between nucleons (protons and neutrons), which involves spin, isospin (quark composition), directionally dependent forces, and non-negligible three-body forces. Second, in a molecule, the energy scales related to the fast, light electrons and the slow, heavy nuclei typically differ by orders of magnitude, making it possible to treat the electrons and nuclei separately, in what is called the Born-Oppenheimer approximation. But in a nucleus, where only nucleons reside, the scale separation tends to be much less pronounced. A third complication is that the attractive short-ranged nuclear interaction tends to coax nucleons to form so-called Cooper pairs, similar to the ones that mediate conventional superconductivity. Because these pairs naturally form a spherical shape, pairing and deformation compete. Which one wins out depends on how a nucleus’s particular combination of protons and neutrons occupy their respective energy shells [3].One standard theoretical framework for analyzing deformed nuclei is density-functional theory, in which the nucleus is allowed to deform its shape in a particular direction to minimize its energy. This choice of a particular direction breaks rotational symmetry, which is then restored by projecting the nucleus’s wave function onto a state of well-defined angular momentum [4]. The process of breaking and restoring symmetries is an efficient way to capture the correlated motion of many-particle systems such as nuclei. However, the mathematical tools—energy-density functionals—used in this approach remain to be rigorously connected to the underlying interaction between nucleons.Ab initio calculations provide an alternative framework. Starting from the interaction between nucleons, ab initio calculations aim to solve the many-body Schrödinger equation using a systematically improvable approximation scheme. The framework has seen substantial progress over the past 20 years [5]. Once restricted to systems of no more than a dozen nucleons, ab initio calculations have been performed for nuclei up to lead-208 [6]. For such heavy systems, it is essential to use approximation schemes whose computational cost scales only polynomially with system size, as opposed to the exponential scaling of more brute-force approaches. One such polynomial-scaling method, coupled cluster, efficiently parameterizes the nucleus’s many-body wave function in terms of particle–hole excitations with respect to a reference state [7]. Another important feature that enables ab initio calculations of heavy nuclei is the reduction in dimensionality obtained by invoking spherical symmetry. Consequently, deformed nuclei, which break spherical symmetry, have become the final frontier for ab initio calculations across the nuclear chart.Sun and his collaborators made two significant advances on this frontier. First, they improved an approach developed by groups working in both nuclear physics and quantum chemistry called projected coupled cluster [8]. In this method, one starts with a deformed nuclear shape, builds particle–hole excitations with the coupled-cluster method, and then projects the resulting, more complicated wave function onto a state of well-defined angular momentum. Although this strategy may seem obvious, as it combines the best of all approaches, the devil is in the details. One needs to formulate the approach so that accurate approximations don’t break the computational bank. While the calculations of Sun and his collaborators are computationally expensive, they are manageable and yield results in good agreement with the available experimental data.The second—and arguably more important—advance is a sensitivity study, which explored how various aspects of the nuclear interaction influence whether a nucleus will be deformed. The calculations used chiral effective field theory ( 𝜒EFT) [9] to derive the nuclear interaction. Working at next-to-next-to-leading order in the 𝜒EFT expansion yielded 17 low-energy constants that specified the interaction. The researchers sampled this 17-dimensional space to determine which parameters most affected deformation. The study revealed a single parameter that has a dominant impact on two signatures of deformation. This parameter controls the short-distance interaction in the so-called 1S0 partial wave—the channel associated with nucleon pairing. The straightforward interpretation is that increasing short-range repulsion in the 1S0 partial wave suppresses the tendency for nucleons to form Cooper pairs and tips the scales in favor of deformation. Interestingly, this same parameter is the main driver for determining how far the excess neutrons stick out beyond the protons in heavy nuclei [6] and for predicting the rate of a speculative nuclear decay called neutrinoless double-beta decay [10].While these results are intriguing, more work remains. The main effect identified by the researchers’ sensitivity analysis seems to inhibit, rather than drive, deformation. Moreover, the long-range part of the nuclear interaction, due to the exchange of pions, was not varied in the analysis because it is well known. But this means it is invisible to the sensitivity analysis. Consequently, it may be that deformation is driven by the least-uncertain aspects of the nuclear force. That would be welcome news for theorists.Ragnar Stroberg is an assistant professor in the Department of Physics and Astronomy at the University of Notre Dame. His research focuses on ab initio many-body methods for studying nuclear structure. After obtaining his PhD from Michigan State University, he held positions at TRIUMF in Canada, Reed College in Oregon, the University of Washington, and Argonne National Laboratory in Illinois.Z. H. Sun, A. Ekström, C. Forssén, G. Hagen, G. R. Jansen, and T. PapenbrockPhys. Rev. X 15, 011028 (2025)Published February 10, 2025Simulations demonstrate that light can be confined within a scattering medium in a way similar to electrons in a disordered metal. Read More »The discovery of an isotope, rutherfordium-252, whose ground state forestalls fission for just 60 nanoseconds, could help theorists understand the cosmic synthesis of superheavy elements. Read More »By incorporating electrical pulses with shapes similar to those of the spikes from biological neurons, researchers improved the ability to train energy-efficient types of neural networks. Read More »Z. H. Sun, A. Ekström, C. Forssén, G. Hagen, G. R. Jansen, and T. PapenbrockPhys. Rev. X 15, 011028 (2025)Published February 10, 2025A new model captures the flow of heat from ocean water into floating ice, providing an important input for efforts to predict future melting in the Arctic.An updated method for detecting trace amounts of gases could allow researchers to identify minuscule amounts of multiple gases in the same sample.A seminal paper by Paul Dirac, who relied on mathematical intuition, laid the foundation for quantum electrodynamics. More Recent Articles »Sign up to receive weekly email alerts from Physics Magazine.Use of the American Physical Society websites and journals implies that the user has read and agrees to our Terms and Conditions and any applicable Subscription Agreement.