Ask Ethan: What are the biggest JWST discoveries so far? – Big Think
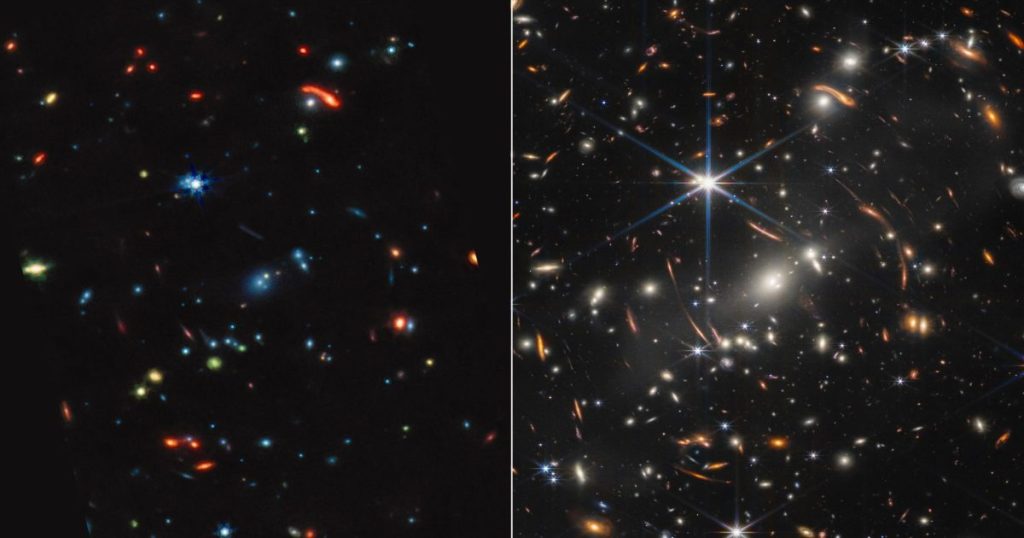
It’s now been more than a full three years since the James Webb Space Telescope (JWST) — humanity’s newest flagship space observatory — was launched into space. Just as the Hubble Space Telescope revolutionized our view of the Universe with its unprecedented capabilities, which it primarily did by showing us what the Universe looked like, JWST is uncovering never-before-seen features and properties of objects throughout the Universe: features and properties that no other observatory, not even Hubble, has ever been capable of. After a little more than six months of pre-science operations, including deployment, alignment, commissioning, and calibration, science operations began in July of 2022.In the 2.5 years that have passed since, we’ve learned an enormous set of new lessons: about exoplanets and their atmospheres, stars, galaxies, star-and-planet formation, cataclysmic events, and much more. And yet, even the people who work professionally (and prolifically) with JWST data can’t keep up with it all. At the 245th meeting of the American Astronomical Society, I was asked a deceptively simple-sounding question by astronomer Dan Coe:“How would you answer the question of, ‘What is the biggest thing we’ve learned or discovered with JWST so far?’”It’s a great question. While JWST observations have led to an enormous number of new record-setting discoveries, there are at least four surprises that have taught us lessons we couldn’t have even anticipated just three short years ago.If you’re someone who focuses on a specific aspect of the Universe — exoplanets, star-formation, ultra-distant galaxies, supernovae, etc. — you’re probably going to pick some discovery in that particular sub-field, because it’s of the greatest interest to you in particular. If you’re interested in:But these discoveries — as profound and compelling as they are — were exactly the types of discoveries that we expected JWST to make.JWST, in fact, was designed with the capabilities it has in order to push precisely these types of frontiers, and these objects represent “more extreme” examples of phenomena that we already knew would exist. However, there’s one critical thing we all need to remember whenever we build a new observatory whose discovery potential (wavelength range, sensitivity, resolution, light-gathering power, etc.) surpasses all previous ones:Every time we look at the Universe in a new way, we discover things we couldn’t have predicted previously.In other words, the Universe is full of surprises that are simply awaiting discovery, and every time we view it with a new transformational tool, technique, or technology, we have a chance to reveal one or more of those hitherto undiscovered pieces of information. With JWST, that’s already happened in four completely independent ways. Here are the four biggest surprise discoveries — and you’re free to choose your favorite, but I’m listing them in reverse-order of my favorites — that JWST has made so far.4.) The discovery of JuMBOs — Jupiter-mass binary objects — within the Orion Nebula.Whenever you have a new astronomical tool whose capabilities represent a vast improvement over all the previous, comparable tools, one of the most important things to do is to revisit the most interesting places that you’ve visited previously with last-generation’s observatories and instruments. JWST has done this with a number of iconic astronomical targets, including:While fascinating, novel features have been spotted when looking at each one of these targets, there’s a profound discovery that sticks out when it comes to the Orion Nebula. This iconic star-forming region, located right around 1500 light-years away, is the closest large star-forming region to ourselves. Within it are a mix of young star clusters, fragmented-and-collapsing gas clouds where star-formation is ongoing, young stellar systems with protoplanetary disks, and neutral material that’s being photoevaporated by the hot young stars shining in their vicinity. When JWST peered inside, we knew we were going to find out additional details about all of these aspects, but we found something new inside that we hadn’t anticipated would even exist in addition.Because of its capabilities — as a uniquely powerful, high-resolution infrared observatory — it was sensitive to not just hot objects like stars and protostars, but much cooler objects: substellar objects like brown dwarfs and even Jupiter-like planets. Many had theorized that there would be some free-floating planets of all masses found within the nebula; likely ejected from young stellar and planetary systems. When it took a look, JWST not only detected an enormous number of Jupiter mass (or super-Jupiter mass) exoplanets, but a significant fraction of them were found in pairs: as Jupiter mass binary objects, or JuMBOs.In fact, of all the free-floating planets — or planets without parent stars — that JWST was able to directly detect, a whopping 9% of them were locked up in binary systems. How did these binary systems come to exist? Were they somehow ejected from a planet-forming system together? Are they examples of “failed binary stars” that didn’t form quickly enough but that remained bound together even as the surrounding material was photoevaporated? These are questions we only know to start asking now, because prior to these JWST observations, we didn’t even know that binary Jupiter-mass objects were something that would, or could, exist in our Universe.3.) JWST uncovered the nature of a long-period gamma-ray burst, and it was merging neutron stars.For a long period of time, scientists have been observing events from the Universe that appear at the highest energies, but only for a brief flash of time. These gamma-ray bursts typically come in two varieties:About 30% of gamma-ray bursts are short gamma-ray bursts, including the famous kilonova event associated with the neutron star-neutron star merger that was seen in both gamma-rays and gravitational waves back in 2017, while about 70% of gamma-ray bursts are long gamma ray bursts, which frequently endure for not just more than two seconds, but up to thousands of seconds.What is it that produces these bursts, which create many of the highest-energy photons naturally produced in the Universe? Theoretically, there are a few options: merging neutron stars, various classes of supernovae, including superluminous supernovae, stellar cataclysms like tidal disruption events, and potential collisions of other celestial objects (such as white dwarfs) with neutron stars. The 2017 event that was seen in both gravitational waves and gamma-rays almost simultaneously — with just a 1.7 second difference between their arrivals — was just a “blip” in gamma-rays: a short gamma-ray burst. But the rapid identification of a kilonova and an associated remnant, localized to a nearby galaxy, seemed to solve part of the mystery: short gamma-ray bursts are created by neutron star-neutron star mergers. So what is it, then, that causes long-period gamma-ray bursts?You’d think it would have to be something different than short gamma-ray bursts, but that puzzle would require superior data to what was available in 2017. That’s where the JWST comes in. If we could find and localize a long gamma-ray burst of sufficient brightness, and then follow up with JWST observations rapidly enough, we could perform spectroscopy and identify what types of signatures are left behind: signatures that would shed light on the nature of whatever cataclysmic event occurred.Well, on March 7, 2023, two NASA observatories — Swift and Fermi — detected a long-period gamma-ray burst that endured for about 200 seconds, definitively in the long-period class. It was also an excessively bright burst: the second most energetic gamma-ray burst observed since they were first observed more than 50 years ago. Shortly thereafter, JWST observed the location where the burst occurred, and did so twice: 29 and 61 days after the burst occurred. Spectroscopy revealed the copious production of several very heavy elements, including tellurium, tungsten, and selenium. The only place known to create these elements is neutron star-neutron star mergers: the same events that trigger short-period gamma-ray bursts.Perhaps all gamma-ray bursts, short or long, have the same type of origin. Why or how? That’s the new frontier, brought about only because of the incredible capabilities of JWST and our willingness to listen to what the Universe tells us about itself.2.) Supermassive black holes must arise independently from the stellar component of the galaxies they occupy.What came first: the supermassive black hole or the stars within a galaxy? Because we know very robustly that one of the ways — perhaps the main way — to make a black hole is through the collapse of a massive star during the end-stages of its life, it made sense to think that black holes would arise from stars. Even though supermassive black holes have grown to very large masses here in the late Universe, they all must have originated from much smaller, lower-mass seeds. When we compare the mass of a supermassive black hole, today, to the stellar mass (i.e., the total mass in the form of stars) of the galaxy where the supermassive black hole resides, we find that even the most massive black holes can only be about 0.1% of the galaxy’s total stellar mass.When we looked at early supermassive black holes prior to the JWST era, we found that they were very large in mass: up to around a billion solar masses, already, when the Universe was just 5% of its current age. But these were still occurring within the brightest, most massive early galaxies we were finding. One “slam dunk” find that was going to arrive in the JWST era was that we’d be able to find much fainter, lower-mass galaxies at these very early times (or even earlier), and that if they exhibited X-ray activity, we’d be able to “weigh” the supermassive black hole just as JWST could “weigh” the stellar mass content of the host galaxy.By late 2023, the chicken-and-egg question was answered for these supermassive black holes. With an assist from gravitational lensing in many cases, galaxies with very low stellar masses — as low as right around 10 million solar masses worth of stars — were copiously discovered. In particular one faint, lensed galaxy, UHZ1, was right at that low value, with only about 10 million solar masses for its stellar mass.But when the X-rays were measured from this galaxy, a mass estimate for the supermassive black hole could be obtained, where it weighed in at 9 million solar masses. For this young, distant object, whose arriving light was emitted a spectacular 13.2 billion years ago, the black hole wasn’t ~0.1% the stellar mass of the galaxy, but right around ~100% the stellar mass of the galaxy.In fact, now, in the JWST era, ratios at great distances are often greater than 0.1%, reaching 1% or 10% frequently, with one remarkable example where it’s all the way up at 100%. These “overmassive” black holes teach us that the black hole must have formed independently of the stars within it, perhaps from the direct collapse of collections of converging streams of cold gas. Supermassive black holes came first, and the early stages of galactic assembly involve stars forming in collections around them.1.) An “asteroid belt” and a “Kuiper belt” might not be defining features in most planetary systems.If you were to rewind the clock back to early 2022, you would have found that the leading thought about star systems is that they would have contained:We find this in our own Solar System, but we also assumed that the physics would be the same: that you’d form a circumstellar disk that would fragment to form planets, and that where the temperature crossed a critical threshold, where various volatiles would be in either the gaseous or solid phases, you’d wind up with belts of solid material.And then we imaged the Fomalhaut system: the youngest star system to Earth that’s incredibly bright and close, and that also has a debris disk around it. What we found was completely unexpected: the presence of not two, but three belts of material around it. Somehow, there wasn’t just an asteroid belt analogue and a Kuiper belt analogue, but an “intermediate” belt in between them. Our default picture was, to the surprise of everyone, not the default in the cosmos at all.Then we imaged a slightly older star that still has a debris disk around it: the even brighter star Vega. Only slightly farther away and a few hundred million years older than Fomalhaut, Vega’s disk happens to be oriented practically face-on to us here on Earth. And yet, when we turned JWST to examine Vega’s disk, we got a different, huge surprise: a smooth, almost featureless disk of material greeted us. There were no gaps, no belts, and no evidence for planets. In fact, the most striking feature of a slightly less-bright “ring” of material near the outer edge of the inner disk is only consistent with a planet whose maximum mass is about 6 Earth masses, or less than 40% the mass of Neptune.Scientists are now examining the idea that Vega might struggle to form planets because of its very rapid rotation, and that perhaps rapidly rotating star systems might not form features like planets or belts at all. Just three years ago, we thought that two belts — an asteroid belt and a Kuiper belt — would be the most common configuration of belts in star systems throughout the Universe. Now, in the JWST era, we know that star systems can have two belts, three belts, or perhaps even zero belts. Which one is the most common? Are systems like ours normal, common, or rare in the Universe? Are there other options; how many belts can a star system have?This, to me, is the most revolutionary discovery of the JWST era. It didn’t occur because we thought it might be there; the discovery occurred because we dared to build an observatory with JWST’s unprecedented power. The payoff — including the surprising payoff of discovering something we never expected — simply arises by listening to what the Universe tells us about itself when we ask.Send in your Ask Ethan questions to startswithabang at gmail dot com!
Source: https://bigthink.com/starts-with-a-bang/biggest-jwst-discoveries/